This article is part of “The New Nuclear Age,” a special report on a $1.5-trillion effort to remake the American nuclear arsenal.
Within every American nuclear weapon sits a bowling-ball-size sphere of the strangest element on the planet. This sphere, called a plutonium pit, is the bomb's central core. It's surrounded by conventional explosives. When those explosives blow, the plutonium is compressed, and its atoms begin to split, releasing radiation and heating the material around it. The reaction ignites the sequence of events that makes nuclear weapons nuclear.
In early nuclear bombs, like the ones the U.S. dropped on Japan in World War II, the fission of plutonium or uranium and the fatal energy released were the end of the story. In modern weapons, plutonium fission ignites a second, more powerful stage in which hydrogen atoms undergo nuclear fusion, releasing even more energy. The U.S. hasn't made these pits in a significant way since the late 1980s.
On supporting science journalism
If you're enjoying this article, consider supporting our award-winning journalism by subscribing. By purchasing a subscription you are helping to ensure the future of impactful stories about the discoveries and ideas shaping our world today.
But that is changing. The country is modernizing its nuclear arsenal, making upgrades to old weapons and building new ones. The effort includes updated missiles, a new weapon design, alterations to existing designs and new pits. To accomplish the last item, the National Nuclear Security Administration has enacted a controversial plan to produce 50 new pits a year at the Savannah River Site in South Carolina and 30 pits a year at Los Alamos National Laboratory in New Mexico, the birthplace of the bomb. The first pits will be designed for a weapon called W87-1, which will tip the new intercontinental ballistic missile, called Sentinel. After that the complex will produce pits for other bomb designs.
Not everyone believes this work is necessary. Pit production foments controversy because it's costly and potentially risky and because the existing pits might still work for a while. The physics of plutonium is complex, and no one knows when the original pits will expire. The details of how the pits are made and how they work are among America's most closely guarded secrets. Yet in June 2023 Los Alamos officials invited a group of journalists to tour the facility for the first time in years.
We were there as the lab and the broader National Nuclear Security Administration Complex were embarking on a charm offensive to support the new plutonium work. They have to win over the tax-paying public and recruit some 2,500 new employees for the job. Some of those workers must do high-hazard work that requires expertise the country has largely let slip since the last days of the cold war. Back then, many thought the world was heading toward disarmament, and the skills necessary for a nuclear resurgence seemed unlikely to be called for. That's not quite what happened. Instead China is rapidly growing its nuclear arsenal, and Russia, at war with Ukraine, touts new missile tests and its own nuclear modernization. The U.S. is doing the same. The world order feels fragile; the renewed focus on nuclear weapons threatens to create a 21st-century arms race and an increased reliance on the shaky peace that nuclear weapons may or may not help keep.
Much of the plutonium work at Los Alamos takes place in a building called PF-4, which is located south of town in a part of the lab complex called Tech Area 55. It is one of the most highly guarded parts of the lab. Before our tour, we're told to survey our hands, forearms and ankles for scrapes or scratches into which radioactive contamination could slip. Onto these abrasions we're instructed to place technical protection: Band-Aids. The message is repeated within the building by a sign that instructs those entering to “occlude your wound.”
A jarring mix of upbeat friendliness and deadly seriousness greets us at the facility. A cheerful wood sign outside the building welcomes visitors, and security guards fist-bump as we walk in. But those same guards wear long guns, and their eyes swivel tightly as we pass through a TSA-like portal into the bowels of the building. A security force will follow the group—who have been parted from phones, cameras and recorders, as well as all metal, nylon and polyester—the entire afternoon.
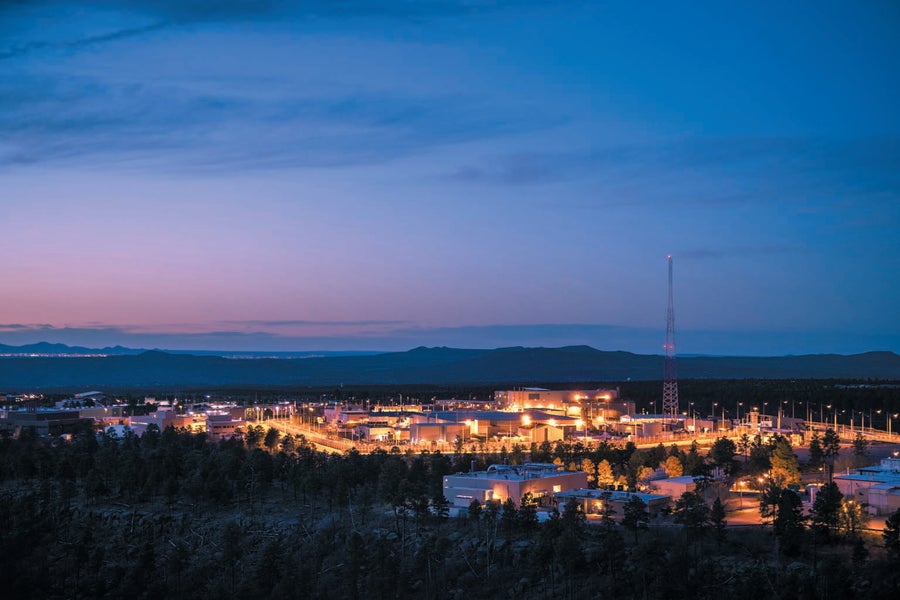
At Los Alamos’s Plutonium Facility, in a building called PF-4, the U.S. plans to produce new plutonium pits for a renewed nuclear weapons program. Credit: Los Alamos National Laboratory
Once we're past the initial screening, we step into an airlock with yellow doors on either side of a capsular room. Only one door can be open at a time to keep potential radiological contaminants trapped within PF-4. After we pass through without setting off the alarm, we put on anticontamination lab coats—color-coded red for visitors without security clearance and yellow for the people who belong here. We scrunch disposable booties over our shoes (the security guards get camo shoe covers). Safety goggles slip over our faces, and radiation badges are clipped to our fronts, measuring the invisible energy and particles that whip through them. All plutonium work has been paused for the day to conceal the classified details of pit production.
Element 94, as plutonium is also known, is rare. Dying stars produce a small amount during their last, hot gasps, but that star stuff had decayed nearly out of existence before Earth formed. The planet has made a bit of its own: in what is now Gabon, Africa, algae concentrated natural uranium over the eons, forming a natural fission reactor that produced four tons of plutonium. That plutonium, too, has since decayed away. Scientists inferred the existence of the natural nuclear reactions from the ratio of uranium isotopes that were left in modern times.
The plutonium used for weapons exists only because people made it. In 1940 scientists used a particle accelerator at the University of California, Berkeley, to bombard an isotope of uranium (which has 92 protons per atom) with nuclei of deuterium (a proton and a neutron stuck together). That created neptunium (93 protons per atom), which conveniently decayed into plutonium with its 94 protons per atom. Thus, one of the most efficient ingredients for making a nuclear weapon was born. It's easier and cheaper to make enough plutonium for a weapon than it is to produce enough enriched uranium, the only other element used to sustain a fission chain reaction in nuclear weapons. And fission is what achieves the pressures and temperatures necessary to ignite fusion in the secondary part of the bomb.
Plutonium's genesis was repeated in reactors for decades. In fact, scientists made so much that no new plutonium is required for the new pits at Savannah River and Los Alamos—the current supply can be repurposed, reshaped, reborn.
None of those actions, though, will be simple because plutonium is not simple. Joseph Martz, a scientist in Los Alamos's materials science and technology division, has spent his career mapping the specifics of that complication. Martz started working with plutonium while he was still in college, manipulating it within protective glove boxes that shield workers from radiation. He's never forgotten the first time he touched element 94. Even through thick gloves and from behind glass, he could still feel it: the kilogram of material in his hand was warm. “I remember actually being a bit afraid,” he says. “I had almost a terror reaction.”
Since then, his fear has given way to fascination with the substance. There is plenty to be fascinated by. It's pliable and compliant in some conditions and delicately brittle in others. When it's a liquid, melting around 650 degrees Celsius, it's the most viscous of all the elements, dripping languidly. If you heat it in its solid form, sometimes it expands, and other times it contracts. It's reactive with air, swiftly shifting its appearance from a silvery metal to a rainbow spectrum of tarnish. When it solidifies, it expands, like water, and its length and density change without much provocation. Its most famous trick, of course, is its propensity for radioactive decay, through which it transforms itself out of existence.
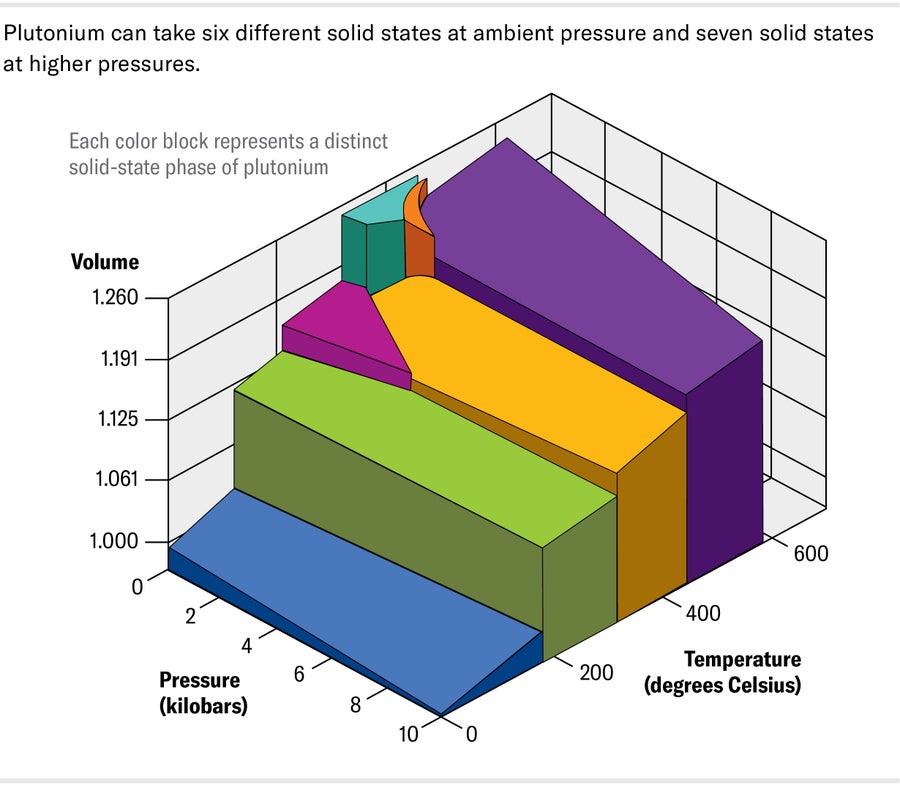
Credit: Modified version of a chart from “New Pressure-Temperature Phase Diagram of Plutonium,” by J. R. Morgan, in Plutonium 1970 and Other Actinides: Proc. 4th Int. Conf. on Plutonium and Other Actinides. Edited by W. N. Miner. Metallurgical Society of the American Institute of Mining, Metallurgical, and Petroleum Engineers, 1970. Adapted with permission from The Minerals, Metals & Materials Society
This tendency is also what makes it so dangerous. Inhaled plutonium decays in the body, releasing alpha particles (helium nuclei) that can wreak havoc. The isotope plutonium 238, used as a heat and power source but not in weapons, exhibits other strange behaviors. “If you spill it in the laboratory, it will move around on its own,” Martz says. The oomph from a plutonium atom's decay sends it shooting across a surface. “It can get everywhere,” he adds.
Plutonium's strangeness comes from its arrangement of electrons. The element occupies the part of the periodic table where atoms' “5f subshell” begins to fill. That's relevant to plutonium's behavior because the “f” electrons reside in narrow energy bands that overlap, allowing the electrons to slip between the bands easily. When they do, Martz says, “the nature of those ‘f' electrons changes behavior dramatically.” Change the temperature, for instance, and some of the electrons bond with nearby atoms “in very complex shapes,” Martz says. The combinatorics mean that plutonium comes in six different solid phases, each with its own crystal structure and strange behavior.
It's taken scientists decades to discover all of this. “What we know today to be the complexity of plutonium was not known to the Manhattan Project scientists,” Martz says. For years those secret scientists didn't actually have any plutonium to study—it had to be painstakingly produced. “Almost everything was theoretical,” says Alan Carr, senior historian at Los Alamos. “In lieu of the actual material, they'd have chalk and chalkboards and notebooks.” The first full gram of element 94 arrived on Los Alamos's mesas in April 1944. The substance had already perplexed the researchers. When they took basic measurements of characteristics such as density, they saw huge variations. Eventually they were able to make their first hemispheres of plutonium metal—protoversions of the pits of today, the size of a golf ball. Yet when they came to the lab the next day ready to experiment, they found that the hemispheres had cracked because their properties and dimensions had shifted. “It was maddening,” Martz says.
A breakthrough came later in 1944, when one Manhattan Project scientist suggested that combining plutonium with another element to make an alloy might stabilize it in a phase that was workable. The problem was, they didn't know which element might work. According to a historical document Martz discovered, the scientists had a very scientific method for deciding what substances to try: “Whatever we found in the cupboard,” they wrote. Eventually they discovered that gallium did the trick. It's still used in pits today.
In the excitement of these early scientific discoveries, the point of the work would sometimes get lost: it was all in the service of creating a deadly superweapon. In 1945 the U.S. dropped a uranium fission bomb on Hiroshima and then sent a plutonium bomb—essentially a pit encased in explosives—to devastate Nagasaki. The bombs killed tens of thousands of people immediately and more after the fact. As Manhattan Project physicist I. I. Rabi had feared, according to a quotation in the 2005 book American Prometheus, “the culmination of three centuries of physics” was a weapon of mass destruction.
Soon after the war, production of plutonium pits migrated to a facility outside Boulder, Colo. Called Rocky Flats, it could churn out thousands of pits a year—a level of productivity perhaps enabled by its violation of environmental regulations, which in 1989 resulted in a federal raid and then a permanent shutdown. “The public wasn't considered at the time,” says Bob Webster, deputy director of weapons at Los Alamos. Not long after, as a result of a testing moratorium and treaty, the country's nuclear weapons complex underwent another phase shift. Scientists and engineers had always tested weapons in the easiest way possible: by blowing them up. If they exploded as expected, officials froze the design and made more clones of that weapon. The researchers never had a true understanding of why everything worked or what might cause it not to—or of how both those things might change over time.
But in 1992 President George H. W. Bush announced a moratorium on nuclear testing. Sig Hecker, at the time director of Los Alamos and now a professor at Stanford University, was in Washington, D.C., when he heard the announcement. “I came back to Los Alamos and told our people, ‘Look, the world has just changed,'” he says. They were going to have to maintain the stockpile by understanding its physics without testing it. That task has proved particularly complicated for the plutonium pits, which are all now decades old. Because plutonium was first synthesized only 80 years ago, no one's been able to observe how it behaves as its life wears on past that point.
How aging affects a pit is the subject of contention, but some things are certain: As the plutonium atoms in a pit decay, their products damage the crystal structure of the plutonium that remains, creating voids and defects. These decays also contaminate the pit with helium, americium, uranium and neptunium, among other things. In 50 years a kilogram of plutonium will amass around 0.2 liter of helium. As pits change, their performance and safety in any conditions—including just sitting on a shelf—become questionable. Pavel Podvig, a senior researcher at the United Nations Institute for Disarmament Research and a researcher with the Program on Science and Global Security at Princeton University, who has questioned the motivation for modernization, concedes, “If you maintain the arsenal, at some point it would be safer to have new pits.”
Scientists still don't know the lifetime of a plutonium pit. JASON—a clandestine group of scientists that provides advice for the government—first projected in 2007 that the pits would last decades longer, implying no production program was necessary. But it changed its stance in 2019, stating, “We urge that pit manufacturing be re-established as expeditiously as possible in parallel with the focused program to understand [plutonium] aging.” The National Nuclear Security Administration's own studies have suggested the pits will last at least 150 years but also that their degradation could result in surprise defects. And scientists may never know exactly what those defects do or how they would affect an explosion because the ostensible point of nuclear weapons is to never use them.
So far restarting American pit production is proving challenging. Los Alamos's efforts are at least a year behind schedule, and Savannah River's are more like five years delayed.
The Defense Nuclear Facilities Safety Board and other critics have claimed that PF-4 isn't resilient enough against the kind of earthquake geologists now know could occur in Los Alamos. Such significant shaking and the fires it could cause, the board alleged at a hearing last year, could result in plutonium contamination that reaches the public. Inside PF-4 our tour group encounters a poster laying out the lab's Seismic Analysis of Facilities and Evaluation of Risk, also known as SAFER, a program that has resulted in upgrades to the building itself and the equipment within. In 2022 the safety board deemed this modernization to be still not quite good enough.
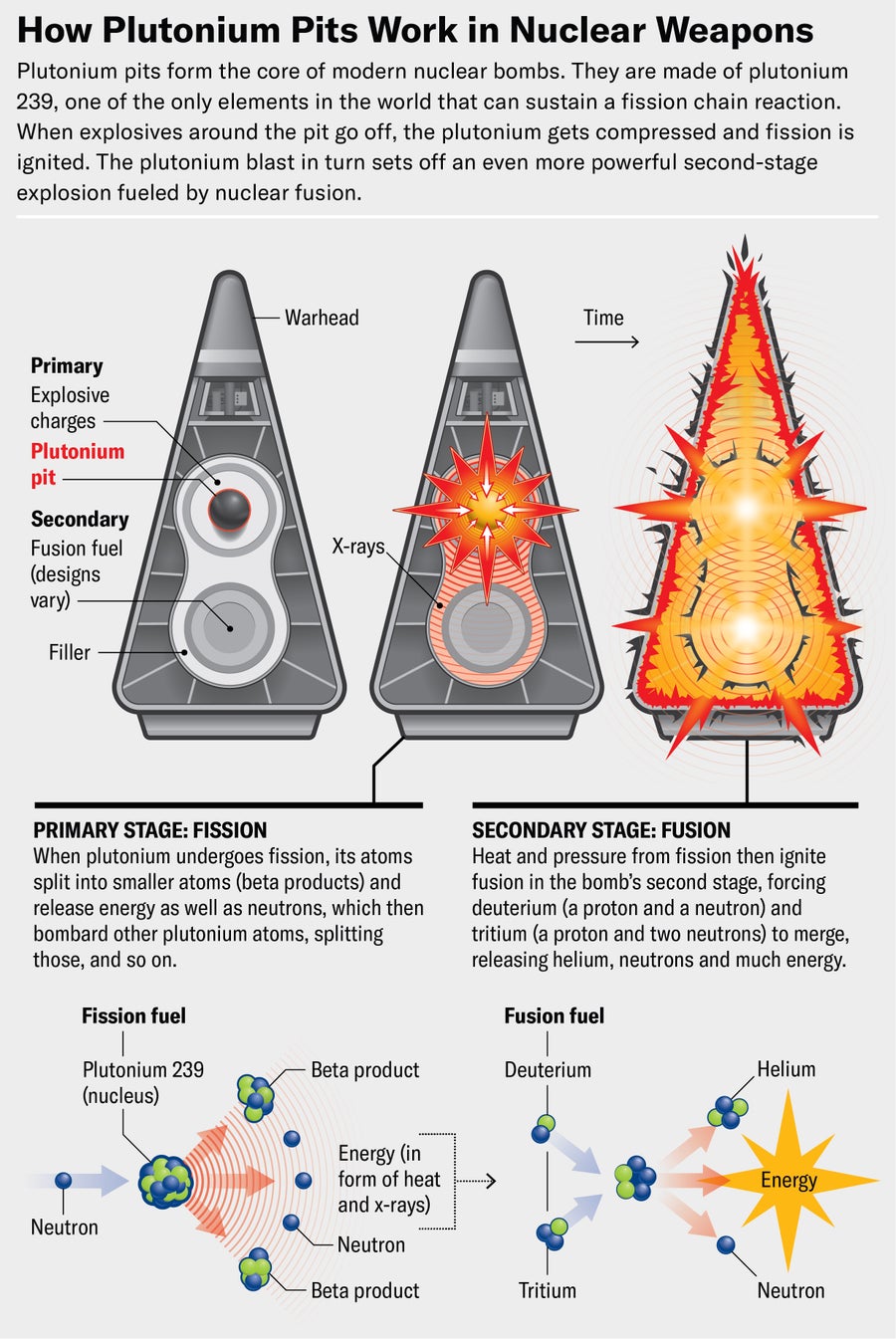
Credit: Nick Bockelman
Some at the lab disagree, including Matt Johnson, head of pit production, who's leading us through PF-4. “If there is an earthquake, this is where I want to be,” he says, gazing at the poster. Other safety concerns have come up recently, though. In May the National Nuclear Security Administration released an investigation about four 2021 incidents: one criticality safety violation, one breach that resulted in skin contamination for three workers, and two flooding events that sent water toward fissionable materials. The agency determined that the contractor that manages Los Alamos had violated safety, procedural, management and quality-assurance rules.
Webster, also on the tour in his official yellow lab coat, says the lab and its workers take safety seriously but admits problems are inevitable. “We will always have issues at the lowest level,” he says. If everything started going perfectly, he says, they would lower the limit of what counts as a safety violation so people would still get practice reporting. But, he notes, the cutoff is already low: “Rooms would get shut down if they were as radioactive as Fiestaware.”
Those rooms, dedicated to various parts of the pit-production enterprise, all have one thing in common: they're full of glove boxes, the radiation-protective equipment inside which workers manipulate plutonium. Long gloves are sealed to openings in glass so sheathed hands can touch samples safely. The gloves themselves have dates written on them so workers know when to replace them. Everyone wears two pairs. The glass windows are surrounded by a metal box that looks like it could be made of plutonium: dull silver with smooth, rounded corners, which are easier to clean and harder for particles to lodge in. “You could eat off this,” Webster says. “Not in here,” he adds.
On the tour, we are forbidden from setting our notebooks down lest potential contamination stick to them. Should we drop them, a radiological control technician—who has been following us the whole time and scans our hands and feet for radioactivity anytime we leave a room—would measure each page before returning them.
In some places the glove box windows are covered by aluminum foil, blocking classified material from our view. Above these boxes, in every room, there is a kind of trolley system that workers use to move plutonium from room to room. In some rooms, radioactive waste is packaged and waiting to go to a storage facility, with the dosage one might receive from standing near it written on the ground. We are never allowed to forget that this is a dangerous place.
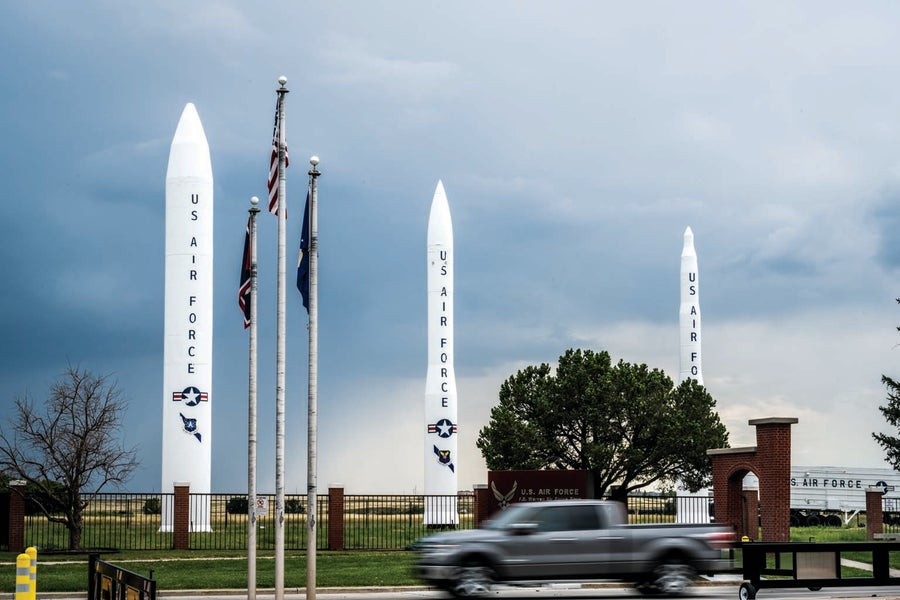
Three intercontinental ballistic missiles—the Peacekeeper, the Minuteman III and the Minuteman I—are displayed at F. E. Warren Air Force Base in Wyoming. Credit: Nina Berman
The workers who make pits face these risks every day. To do their jobs, they must first recover and purify plutonium from the old material. Then they “cast,” or shape, the plutonium into pieces. Once cast, the pieces must be fitted together into a whole. Standing near the place where that happens is the first woman to ever assemble pits, whose name we cannot publish for security reasons. She assembled her first pit in 2013 (between 2007 and 2013 the lab produced 31 pits). Today putting a pit together takes her 30 minutes to an hour. “Everything is by touch, by feel,” she says. She likes the work, some of which happens in a glove box that's two stories tall. “It's peaceful in the glove box,” she says.
Once she or another worker has finished a pit, it gets micromeasured and constitutionally scrutinized to confirm it meets specifications. If it gets a stamp of approval (with a literal stamp, shaped like a diamond), it will go to the Pantex facility in Texas to be placed in a nuclear warhead. In the years to come, if all goes as planned, that process will happen at least 30 times a year here.
All of this effort and investment is being made in the hopes that the pits never serve their active purpose. The U.S., like all nuclear nations, stockpiles weapons in a delicate game of deterrence, the idea being that the existence of our equally or more capable weapons will stop others from using theirs. In this strategy, the pits' true purpose is to sit idly as a threat. But for the strategy to work, the country must be willing to follow through on that threat.
As we leave PF-4, permanently installed instruments once again scan our hands and feet for radiation. After that, in an airlock, a full-body scanner sniffs for alpha, beta and gamma radiation on our bodies. Even though contamination is unlikely, we sigh with relief when the all-clear comes.
We go back to our lives, where we can easily forget about plutonium pits. After the cold war many Americans got used to these weapons. “At some point it became so normal it was forgettable,” says Idaho State University nuclear historian Sarah Robey. The fear people feel when confronted with plutonium has degraded over time. But the atomic age is renewing, and we will all have to grapple afresh with the coiled terror of these powerful weapons.