Scientists are fond of saying negative results are just as important as positive results, but after several decades of not finding something, researchers can be forgiven for feeling impatient. Back in the 1990s, experiments began trying to detect the particles that make up dark matter, the ubiquitous yet untouchable invisible material that apparently fills the cosmos. Since then, physicists have found more and more evidence that dark matter is real but not a single sign of the stuff itself. A new version of the long-running XENON experiment that started up late last year aims to finally break that pattern.
One of physicists' best guesses about the identity of dark matter has long been that it is made of particles called WIMPs—weakly interacting, massive particles. These elementary bits of matter could be anywhere between the mass of the proton and 1,000 times the mass of the proton, and they would interact with regular atoms only through gravity and the weak nuclear force, which governs radioactivity. But over the years, as experiment after experiment failed to find anything, some of the enthusiasm has faded. “You do start to scratch your head and think maybe that was the wrong horse to bet on,” says Rafael Lang, a physicist at Purdue University, who has been working on the XENON experiment at the Gran Sasso National Laboratory in Italy for more than a decade. For now, though, Lang says he is keeping his money on WIMPs, pointing out that the experiments have falsified many of the theories predicting what WIMPs might look like but certainly not all. “If you believed in WIMPs 10 years ago, only half of those WIMPs have been ruled out,” he says. “The other half are still alive.”
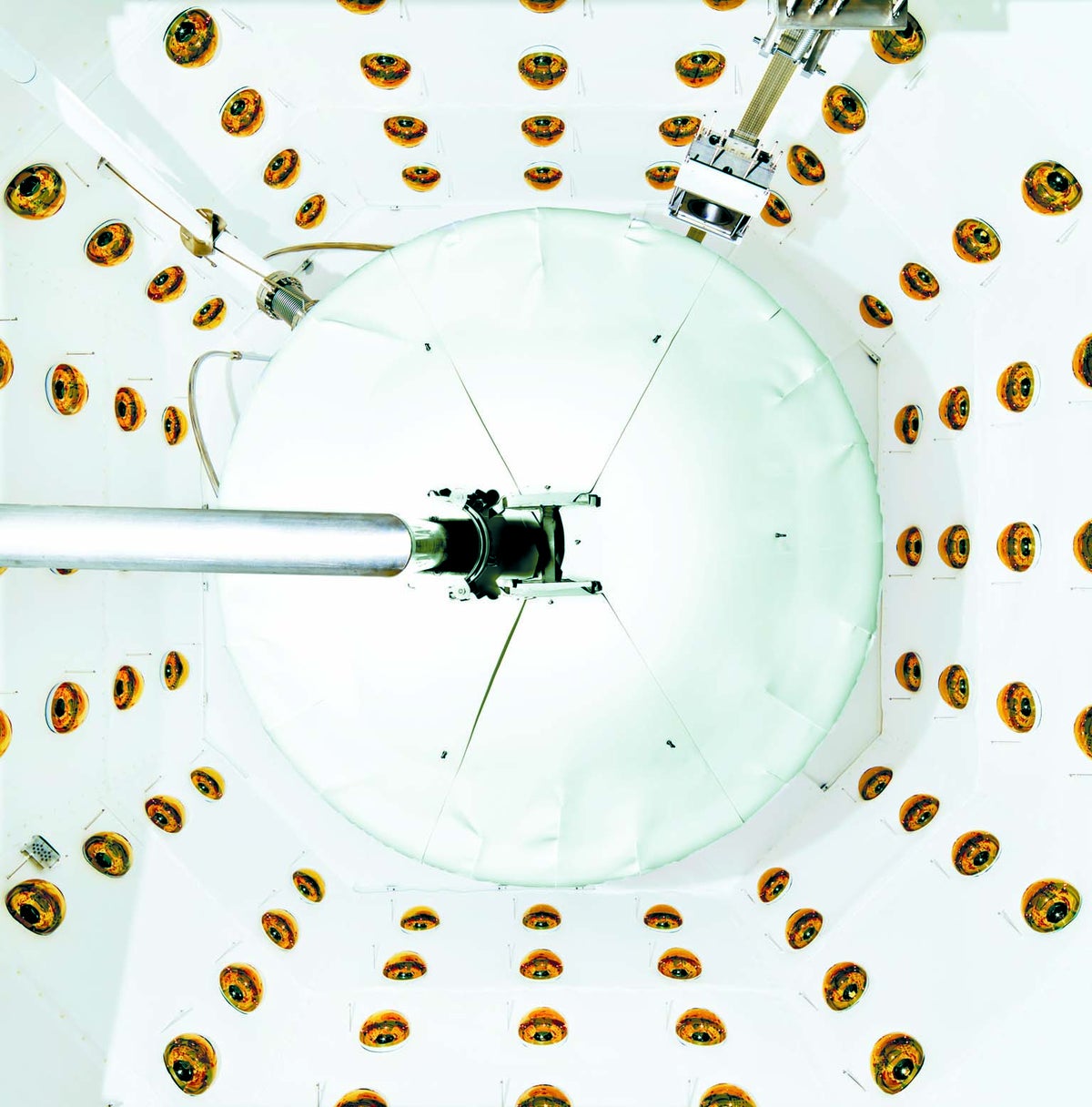
Onion Layers: The XENONnT experiment is structured like an onion, with multiple layers to keep out extraneous particles that might imitate dark matter. The first is the mountain on top of the underground Gran Sasso lab, which stops most cosmic rays from space that might contaminate the results. The next layer is a roughly 10-by-10-meter water tank that encircles the inner contents, blocking most of the particles that are released from radioactivity in the walls and rock surrounding the lab. In the tank is the neutron veto system, which will also be filled with water, shown here from the inside. Nestled within is a white cylindrical outer cryostat—essentially a thermos—that contains an inner cryostat. The inner cryostat surrounds the detector itself, which is filled with liquid xenon.
Credit: Enrico Sacchetti
On supporting science journalism
If you're enjoying this article, consider supporting our award-winning journalism by subscribing. By purchasing a subscription you are helping to ensure the future of impactful stories about the discoveries and ideas shaping our world today.
Of course, so are many other dark matter candidates. Another top contender is the axion, a much lighter theorized particle that has lately morphed into a fluid category of possibilities called axionlike particles. Some scientists are excited about the idea that dark matter may be a composite particle—a conglomerate of “dark quarks” and “dark gluons” that stick together just like regular quarks and gluons to create “dark nuclei.” It is also possible that dark matter is not a particle at all. One idea still in the running is that the missing matter is made of primordial black holes that formed soon after the big bang.
The newest version of the XENON experiment, XENONnT, began collecting data last year. Its goal is to catch dark particles on the very, very rare occasions when they might bump into regular atoms. The atoms in this case are xenon—8.3 metric tons of it, kept in liquid form in a giant vat buried under a mile of rock to shield it from cosmic rays and other contamination. Xenon, with its 54 protons and electrons and even more neutrons, is a good, dense target for dark matter to bump into. If an exotic particle were to hit a xenon nucleus, it might send the nucleus or an electron flying through the liquid, creating a flash of light that photomultipliers on the top and bottom of the vat can detect. This latest iteration of the experiment contains four times more xenon than the previous version, which means it is four times more likely to see a signal.
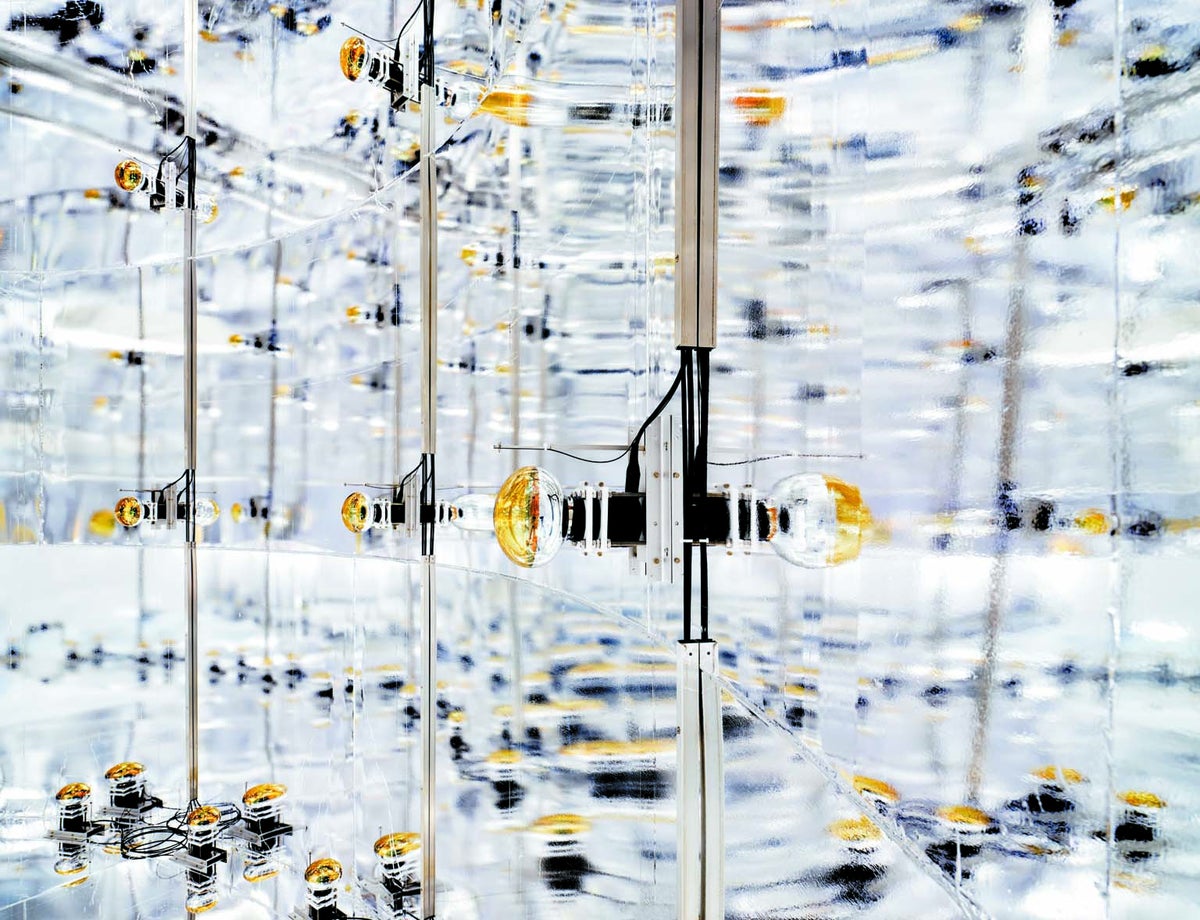
Mirror Image: Photomultiplier tubes line the water tank, which is covered in reflective foil that creates a double image. The optical instruments are sensitive enough to detect a single photon released by a particle interaction. In this case, the tube is designed to see the signals of muons, which may infiltrate the experiment as cosmic rays. Slightly different photomultiplier tubes line the neutron veto layer and the innermost detector.
Credit: Enrico Sacchetti
Other upgrades include improved purification of the xenon and better systems to detect cosmic rays and trace amounts of radioactive elements in the experiment and its housing that could masquerade as dark matter signals. “Every nut and bolt on the detector is custom handmade from carefully selected materials,” Lang says, “because if you just buy a stainless-steel bolt at the hardware store, it's too radioactive for what we need.”
To the outside world, the years of painstaking work without the reward of a discovery might seem disappointing, but physicists see it differently. “If you're judging by whether it has detected dark matter, they haven't, but in the eyes of the community it is a dramatically successful experiment,” says theoretical physicist Dorota Grabowska of CERN, who is not part of the project. Its success, she says, lies in the many possibilities it has ruled out and the ever increasing sensitivities it has achieved.
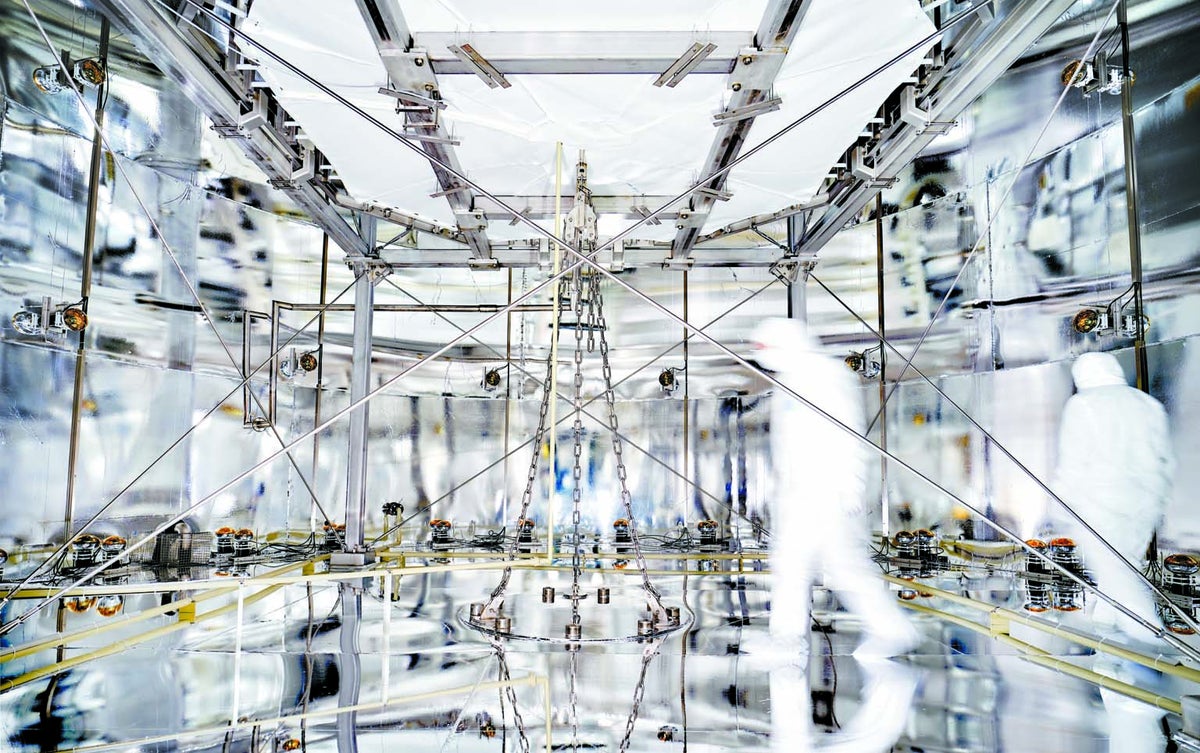
Final Check: A technician makes a last sweep through the unfilled water tank. The XENONnT experiment will run for five years before reaching its design sensitivity. At that point, it will have either discovered WIMPs or ruled out more than two thirds of the theoretical WIMP possibilities still on the table. A similar experiment called the LUX-ZEPLIN (LZ) project is being carried out in South Dakota. The two use slightly different setups and will be important checks for each other if either sees a signal of new particles.
Credit: Enrico Sacchetti
Now the search for WIMPs stands at a turning point. In the relatively near future, underground dark matter experiments will have searched most of the theoretical landscape they can reach. If they have not found WIMPs, it may mean they do not exist, or it may simply mean they take some form that has managed to escape notice. But scientists are resourceful—they can think of new ideas for what dark matter might be and new ways to search for it faster than they can build detectors. “There is a lot of excitement and creativity around identifying new ways to detect dark matter candidates,” says theoretical physicist Tongyan Lin of the University of California, San Diego. One idea she works on involves using crystals to catch dark particles. In crystal form, elements such as silicon might register an interaction with dark matter at lower energies than traditional detectors, opening up a new avenue for discovery.
Although dark matter has proved more elusive than some had initially hoped, physicists are far from giving up. “A lot of people have a view of science that is like Star Trek,” says theoretical physicist Tim Tait of the University of California, Irvine. “You see something and take out a tricorder and get an answer. But it's actually a very messy process, and you try lots of things until you find something that works. All the things that didn't work were an important part of the process.”