What began as a debate over astronomical measurements is on the verge of becoming a full-blown crisis in how we understand the cosmos. Two data sets—one from the newborn universe nearly 14 billion years ago, the other from stars as we see them today—are yielding contradictory answers to a deceptively simple question: How fast is the universe expanding?
The gap between answers is only 9 percent, but that far exceeds each data set’s estimated uncertainties. Researchers on each side of the gap call it “the tension,” and are digging in their heels about the validity of their observations. This tension is the stuff of scientific dreams—and nightmares. It hints that somewhere, somehow, our understanding of the laws of nature may be fundamentally flawed—with potentially profound implications for physics, and perhaps even the fate of all things.
“If the tension isn’t a fluke and it’s not an error in measurement, it implies we’re missing something in our models,” says Adam Riess, an astrophysicist at Johns Hopkins University and the Space Telescope Science Institute. “Making this measurement for the early universe and then comparing it to today’s is an end-to-end test of the whole story we’ve constructed about the universe. The trouble is, if something definitely doesn’t fit, we don’t know where exactly the story diverges.”
On supporting science journalism
If you're enjoying this article, consider supporting our award-winning journalism by subscribing. By purchasing a subscription you are helping to ensure the future of impactful stories about the discoveries and ideas shaping our world today.
The answer to the question of the universe’s expansion rate is something called the Hubble constant, named after the astronomer Edwin Hubble who discovered in the 1920s that the universe is expanding. Galaxies recede from us at speeds proportional to their distances, going faster the farther away they are. The Hubble constant codifies this relationship between cosmic distances and velocities. But in doing so it reveals much more, making it of interest not only to astronomers but also to cosmologists and physicists. Because the constant represents the expansion rate at any particular moment in the universe’s long history, measuring its value over time provides an expansive view of how the universe evolves over the eons, giving researchers crucial clues to our cosmic origins and future. Somehow beckoned by the void, billions of outward-rushing galaxies also feel the collective gravitational pull of everything in the rearview mirror trying to tug them back. The Hubble constant reflects the sum total of all the stuff in the universe and the forces acting on it—weighing in on whether gravity or the void will ultimately win this intergalactic tug-of-war.
The universe’s contents could eventually reverse the expansion—a scenario called the “big crunch,” in which gravity pulls everything back into an infinitely hot and dense point like the one that birthed the big bang. Or the universe might steadily expand indefinitely, growing ever colder and listless in a “big chill” that offers endless space and time—but ultimately very little to do. Or just maybe the cosmic expansion will dramatically speed up, becoming so unruly that it bucks all its riders. Such an accelerating universe could sunder galaxies, then stars, then planets, atoms and subatomic particles until even the fabric of reality itself splits at its seams in a “big rip” that leaves practically nothing behind. Will the universe end in fire, ice or emptiness? The Hubble constant knows—but until the tension is resolved, the answer is unclear.
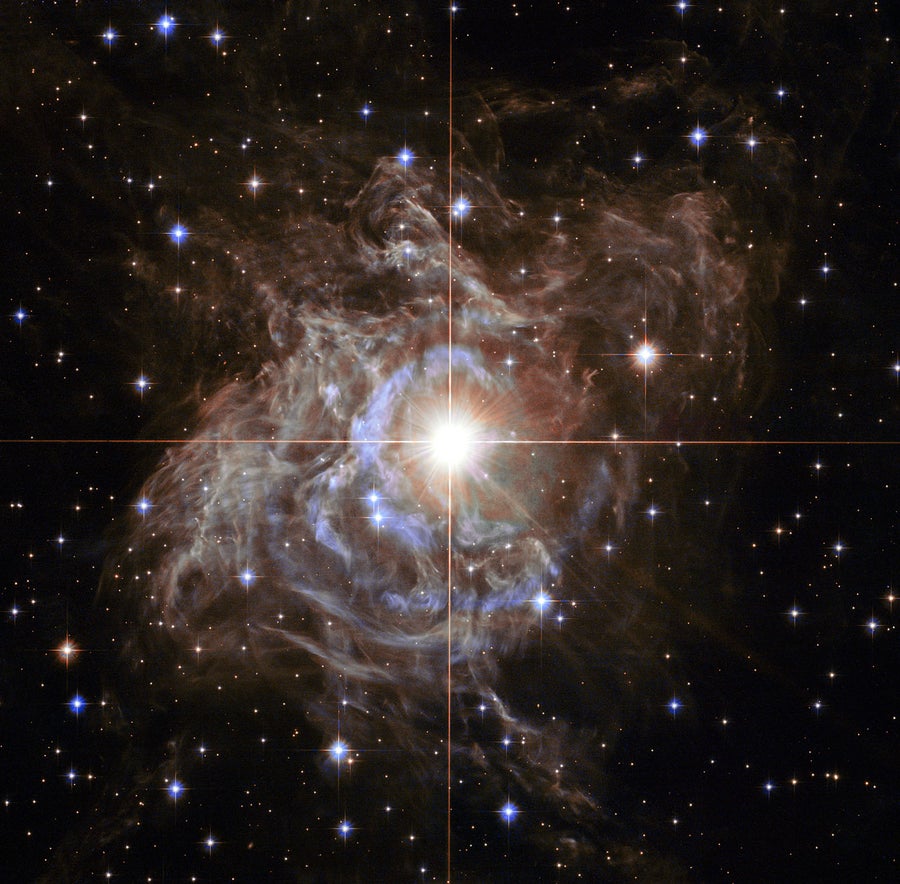
A Hubble Space Telescope image of the Cepheid variable star RS Puppis. Astronomers use Cepheid variables as well as supernovae to measure the expansion rate of the universe. Credit: NASA, ESA and Hubble Heritage Team
Supernova Shoe-Gazing
“This is the most important of the cosmological parameters,” says Wendy Freedman, an astrophysicist at the University of Chicago who has spent her career pursuing the Hubble constant. “It is the anchor because it has the highest impact on the greatest number of things. This is a measurement that really matters.” In the 1990s and early 2000s Freedman led a team that used the Hubble Space Telescope to provide what were then the constant’s best-yet measurements. Others have since been improving on them.
For the past decade Riess has been at the forefront of that effort, leading a team of astronomers who are using telescopes on the ground and in space to further refine estimates of the Hubble constant, in a project called “SH0ES” (don’t ask).
SH0ES’s targets of choice are exploding stars called type Ia supernovae. These exploding stars flash with near-identical luminosity throughout the cosmos, making them ideal “standard candles” to gauge distances to other galaxies. By knowing how bright a type Ia actually is versus how bright it appears to be in their telescopes, scientists can calculate how much space lies between Earth and that far-off stellar cataclysm. They can also measure each supernova’s redshift—the way the expansion of space between the supernova and Earth has stretched the supernova’s light to longer, redder wavelengths. They then estimate the rate of expansion by comparing the redshifts and brightness of many supernovae scattered across the cosmos. To calibrate their supernovae measurements, however, the SH0ES team also uses another standard candle: Cepheid variable stars, which periodically pulsate in relation to their luminosity and offer superior distance measurements in the vicinity of the Milky Way.
Pairing the supernovae and Cepheid data has allowed the SH0ES team to get steadily more precise estimates for the Hubble constant, reducing the measurements’ margin of error from 5 percent in 2009 to just 2.4 percent in 2016. Their latest effort, calibrated using new and improved Cepheid distance data from the European Space Agency (ESA) Gaia spacecraft, reduces the uncertainty to 2.2 percent. Over the years the SH0ES team’s calculations of the Hubble constant have remained remarkably consistent: According to supernovae and Cepheids, the universe is expanding at a rate of 73.5 kilometers per second per megaparsec (about 3.3 million light-years). That is, for every 3.3 million light-years of space between us and another galaxy, the latter will recede from us 73.5 kilometers per second faster.
The tension emerged from independent measurements made by another ESA craft—Planck. From 2009 to 2013 Planck created an unprecedentedly detailed map of the cosmic microwave background (CMB), the afterglow of the big bang’s primordial fireball from when the universe was only 380,000 years old. The Planck team derived the Hubble constant from that bygone era by first modeling the sizes and motions of sound waves that should have rippled through the soup of charged particles that filled the early universe. Next, they compared those estimates with the actual echoes imprinted on the CMB. The comparison provided both the distance to the CMB and the scalar dimensions of its features, allowing the Planck team to clock the primordial universe’s expansion rate at just 67.3 kilometers per second per megaparsec. That estimate, and its remarkable error margin of just 1 percent, hinge crucially on the well-established “standard model” of cosmology—a kludgelike theoretical construct that robustly predicts many observed features of both the CMB and the contemporary cosmos.
“This is like the pediatrician measuring and calculating that your kid will end up being six feet tall, but your child ends up growing to be six and a half feet,” Riess says. “It means something else is going on—maybe your kid had a growth spurt or got hormone injections. In this case, it’s the physics of our best cosmological model providing the growth chart. But who’s to say we actually have that right?”
Having now passed yet another test via Gaia’s Cepheid distance measurements, Riess says, the odds of the SH0ES Hubble constant measurement being a statistical fluke are just one in 7,000. Physicists typically consider a measurement significant when it reaches the one-in-a-million realm of statistical likelihood; at present, the SH0ES results remain short of that lofty standard, but are getting closer all the time. Meanwhile, the Planck team is not budging, either; the validity of its result, team members have consistently said, is practically unassailable.
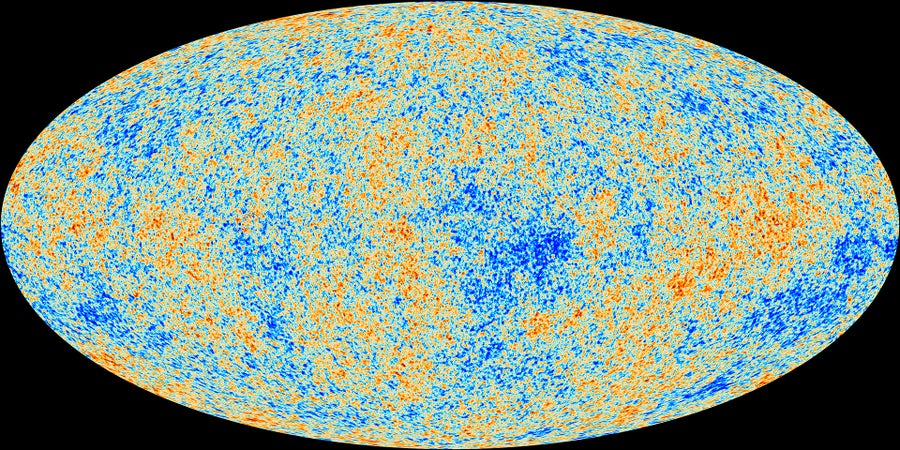
The Planck spacecraft’s all-sky map of variations in the cosmic microwave background (CMB), the oldest observable light in the universe. Estimates of the universe’s expansion rate derived from Planck’s data conflict with those from supernovae and other sources. Credit: ESA and Planck Collaboration
Beyond the Standard Model
This is not the first time the universe’s expansion has flummoxed scientists. In the 1920s the expansion itself came as a shock to most researchers, especially Albert Einstein. Contrary to his preference for a static cosmos, Einstein’s theory of general relativity predicted a universe that would inevitably expand or collapse. To “fix” this he added a new term to his calculations: a sort of antigravity suffusing all of space that could act to preserve universal equilibrium. Einstein first dubbed it “the cosmological constant”—but later allegedly called it his “biggest blunder,” following Hubble’s discovery. Einstein’s initial intuition was apparently vindicated beginning in the 1990s, when Riess and other astronomers found distant type Ia supernovae were dimmer (and thus farther away) than expected. A mysterious “dark energy” seemed to be causing the universe’s expansion to accelerate; perhaps, many physicists speculated, the dark energy and the cosmological constant were one and the same. Measurements from the CMB and other sources rapidly confirmed dark energy’s existence if not its exact nature, resulting in Riess and two others receiving the 2011 Nobel Prize in Physics.
Because its effects would be evenly distributed throughout all of space, as space itself expands the cosmological constant would become more powerful, ramping up the rate of acceleration to produce either a big chill or a big rip as the universe’s ultimate fate. But that boost, it seems, would still fall short of the Hubble constant the SH0ES team and other groups observe in the universe today. So the current tension, Riess speculates, could be due to dark energy not being Einstein’s cosmological constant at all (although he hastens to add such scenarios are not strongly supported by observations of galaxies midway between the CMB and the present). If dark energy is not Einstein’s cosmological constant, it could potentially fuel an even speedier acceleration, easing the tension. In theory, such a nonstandard form of dark energy could also profoundly diminish or even reverse its effects in the future, leaving open the possibility that the universe could still experience a big crunch.
Other speculative explanations exist for the tension, each one another path researchers must follow through the maze of possibilities deciding the ultimate fate of the cosmos. They include as-yet-undiscovered varieties of fast-moving subatomic particles, the influence of hidden “extra” dimensions, or various interactions with dark matter—to name just a few. It could be that more than one type of physics beyond the standard model is at play in the apparent tension between Hubble constant estimates from opposite ends of the universe.
A Conspiracy of Errors?
Then again, some skeptics say, the most likely explanation is simply mistakes made in measurements. The Planck team’s party line, in particular, has been that errors in calibrating Cepheids and type Ia supernovae are probably to blame for the tension.
“We don’t know what the answer is, but there really aren’t any theoretical explanations jumping out at us as very reasonable,” says Lloyd Knox, a Planck team member at the University of California, Davis. “Speaking solely for myself, if I had to place money on anything, I’d still guess the tension is a systematic error in the direct measurement of the Hubble constant [in the modern universe].” For instance, Knox says, the glare from background stars in distant galaxies can contaminate brightness measurements for Cepheids, sabotaging astronomers’ rickety cosmic distance ladder near its base and throwing off dependent measures of greater distances. In contrast, Knox and others note, the Planck team’s derivation of the Hubble constant aligns with multiple and extremely robust independent lines of evidence—such as the large-scale clustering of galaxies and the observed ratios of light elements generated in the first few moments after the big bang. And Planck’s results, Knox says, was also recently validated via follow-up CMB studies using the South Pole Telescope.
Riess argues that time and time again tests performed by SH0ES and other teams have shown background stars are not a significant source of errors in Cepheid measurements. Furthermore, the SH0ES result comes with a wealth of corroborating data all its own: Separate from supernovae and Cepheids, other measurements of the Hubble constant in today’s universe arrive at a value close to the 73.5 found by SH0ES. In 2017 an international team dubbed H0LiCOW (again, don’t ask) clocked the Hubble constant at 72 kilometers per second per megaparsec. They did so by measuring the delayed arrival times of light rays from far-distant galaxies as the rays’ various paths through space were distorted by massive galaxies closer to Earth.
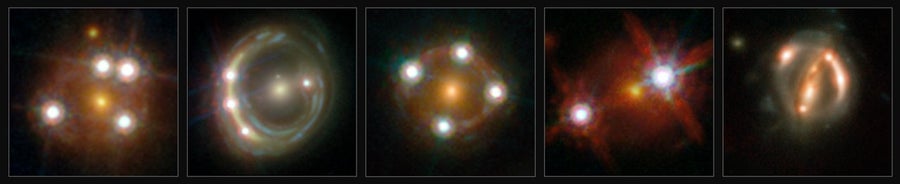
This montage shows light from five background galaxies being distorted by massive foreground galaxies closer to Earth. The distortion causes the background galaxies to appear as multiple images. Scientists in the H0LiCOW collaboration studied these objects to make an independent measurement of the universe’s expansion consistent with earlier estimates based on supernovae and Cepheid variables. Credit: NASA, ESA, Hubble and Suyu et al
That result, says H0LiCOW team member Tommaso Treu, an astrophysicist at the University of California, Los Angeles, is based solely on basic geometry and Einstein’s general relativity—and is thus wholly independent of factors that might sully SH0ES or Planck measurements. “In combination with the SH0ES result, this adds evidence for the tension,” Treu says.
Taken together, Riess considers the evidence supporting his result to be almost overwhelming. For it to be wrong, he says, would require “a conspiracy of errors—multiple errors, one for every approach, that are independent but by some malevolence all the same size and in the same direction. And as Einstein said, ‘God is subtle, but he is not malicious.’”
David Spergel, an astrophysicist at Princeton University and the Flatiron Institute, believes it is time for teams on both sides to acknowledge uncertainties in their data may be greater than previously believed. “Historically, both astronomers and cosmologists have underestimated their errors; I think that’s true for the measurements here, too,” he says.
A resolution may be on the horizon. Spergel is one of the lead scientists planning NASA’s Wide-Field Infrared Survey Telescope (WFIRST), a space observatory slated to launch in the 2020s with a primary goal of studying dark energy. The ESA is planning a similar mission, Euclid, that would complement WFIRST’s studies. These missions could help resolve the tension by clarifying whether dark energy behaves like Einstein’s cosmological constant or something wildly different. NASA’s James Webb Space Telescope, slated to launch as early as 2020, could also provide an avalanche of new observations to constrain the constant, as will Gaia data releases in 2020 and beyond. Around the same time other novel techniques are likely to mature as well. These would rely on surveys of previously inaccessible stellar populations or even observations of gravitational waves from large numbers of colliding neutron stars to obtain additional independent measurements of the Hubble constant.
For now, however, the tension remains—a figurative and literal symbol of how fast our understanding of the universe is accelerating, and how far we still have to go.